Posted By: technopediasite
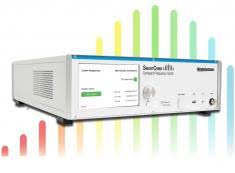
An optical "frequency comb" is a very precise tool for measuring different colors or frequencies of light.Now technology is able to measure the colors & frequency of optical fiber also. This technology also used in telecom sectors.The technology, made possible by recent advances in ultrafast lasers, can accurately measure much higher frequencies than any other tool. Frequency combs are already widely used in metrology laboratories and physics research, and they are starting to become commercially available.
Optical frequency combs rely on the relationship between time obviously a familiar concept and frequency, which is less familiar to most people, but is simply the number of oscillations per unit of time. NIST scientists start with lasers that emit a continuous train of very brief, closely spaced pulses of light containing a million different colors. The properties of the light over time are converted to frequency numbers to make what looks like a comb. Time and frequency are inversely related; that is, smaller units of time (or faster oscillations of light waves) result in larger frequency numbers.Optical frequency combs are central to the development of optical atomic clocks. Researchers are currently working on applications of combs in advanced laser radar, exquisitely sensitive chemical detectors, and secure optical communications systems. Laboratory scientists are using them to measure the energy levels of electrons and probe their dynamics inside atoms. And, thanks to frequency combs, revolutionary advances in our ability to exactly control chemical reactions with lasers are just around the corner.
The graphic below shows how a few different colors of light oscillate over time. This example is greatly simplified, and the specific units are unimportant (in reality the units would be tiny fractions of seconds). The essential point is that the blue waves oscillate much faster than the red waves, and the yellow and green waves are somewhere in between.
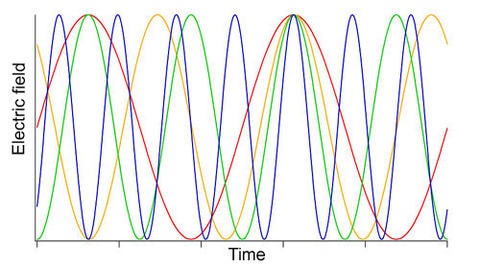
In the past few years, researchers have even used frequency combs to span the huge frequency (and wavelength) gap between radio and light waves, connecting them in ways that were impossible until just a few years ago. This connection goes to the heart of our ability to measure frequency and time, enabling the developing of a new generation of atomic clocks based on optical transitions in atoms. These new clocks use frequency combs to count the oscillations of light produced by optical atomic transitions and convert them to useful electronic signals. The comb technology used in optical atomic clocks is so precise that every one of the colors in a laser pulse train can be exactly linked with one another to produce (and measure) a symphony of hundreds of thousands of pure optical tones.
A simplified graphic of a corresponding frequency comb is shown below. Each "tooth" of the comb is a different color, arranged according to how fast the light wave oscillates in time. The waves that oscillate slowly (red) are on the left and the waves that oscillate faster (blue) are on the right. Frequency is measured in hertz, or cycles per second. An actual optical comb does not begin at zero on left, but at a very high number, 300 trillion hertz.
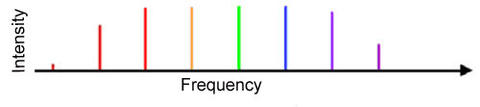
A real optical frequency comb spans the entire visible spectrum of light, and has very fine, evenly spaced teeth. The teeth can be used like a ruler to measure the light emitted by lasers, atoms, stars, or other objects with extraordinarily high precision.
Mode-locking refers to how the laser light is formed into pulses. In all lasers, light is repeatedly reflected within a mirrored cavity. In a mode-locked laser, the peaks of the different colors of light waves coincide at regular intervals, evenly spaced in time. The peaks build on each other to form very short, bright bursts of light, each containing many different frequencies
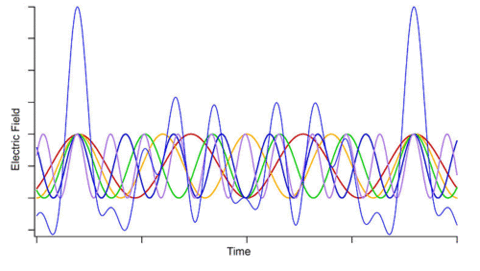
The timing between pulses determines the spacing between the teeth of the frequency comb. NIST scientists use lasers that emit about 1 billion pulses per second. The faster the pulse repetition rate, the wider the spacing between the teeth, making each individual tooth easier to identify.
Finally, the stability of the laser determines the width of the individual comb teeth. A highly stabilized laser produces very fine teeth, enabling highly precise measurements of specific frequencies or changes in frequency. Special crystals, mirrors, and other techniques also are used to make the light waves and comb teeth as perfectly spaced as possible.
How do Frequency Combs Work?
Optical frequency combs are particularly useful because the optical frequencies of comb lines can be determined by two radio frequencies, the repetition rate, and the offset frequency. High-speed photodiodes can readily measure the repetition rate. However, it wasn’t until 2000 that scientists at JILA figured out how to measure the offset frequency in a frequency comb that spanned an entire octave of frequencies. The octave-spanning spectrum allowed them to compare two comb lines at opposite ends of the spectrum with each other. If the offset frequency had been zero, then the two comb lines would have been found to have a frequency ratio of exactly 2. Instead, they found a small deviation, equal to the offset frequency, from this exact ideal ratio.
It turns out that the frequencies of two comb lines with a ratio of approximately 2 can be compared by doubling the frequency of the comb line on the low-frequency side of the spectrum. Frequency doubling is accomplished with second-harmonic generation crystals, which produce an output electric field that is the square of the input field. And, squaring a sine wave produces a sine wave at twice the frequency.
If the low frequency comb line has a frequency of νn = n•Æ’rep + Æ’0, then the comb line that is closest in frequency to twice νn has a frequency ν2n = 2n•Æ’rep + Æ’0. One can easily determine their frequency difference via a beat note that occurs when both illuminate the same photodetector (known as heterodyne detection, identical in concept to the "beating" sound used in tuning a musical instrument). This simple technique yields 2ν2n-νn = 2(n•Æ’rep + Æ’0) – (2n•Æ’rep + Æ’0) = Æ’0 and is known as "self-referencing." The output of heterodyne detection gives a signal that can be used to generate an error signal in a phase-locked feedback loop to stabilize the frequencies of all of the comb lines.
Frequency combs have dramatically simplified and improved the accuracy of frequency metrology. They also are making it possible to build optical atomic clocks, expected to be as much as 100 times more accurate than today's best time-keeping systems. Better clocks will lead to studies of, for example, the stability of the constants of nature over time, and enable improved technology for advanced communications and precision navigation systems, such as next-generation global positioning systems.
Highly accurate measurements of frequencies are also essential for many other advanced fields of science that require the identification or manipulation of atoms or molecules, such as detection of toxic biochemical agents, studies of ultrafast dynamics and quantum computing. As scientists continue to improve frequency comb technology and make it easier to use, it may be applied in many other research fields and technologies, from medical tests in doctor's offices, to synchronization of advanced telecommunications systems, to remote detection and range measurements for manufacturing or defense applications.
What Are Frequency Combs Made
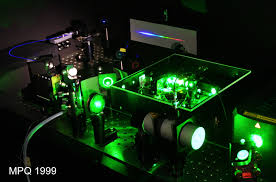
Mode-locked lasers generate optical frequency combs by creating regular trains of incredibly short pulses of light that last anywhere from 20 to 100 quadrillionths of a second (10-15 s), depending on the individual laser. Today, well-stabilized mode-locked lasers, together with associated electronics and a specialized interferometer for comparing comb lines, are sometimes referred to as "Frequency Comb Lasers" or simply "Optical Frequency Combs." If such a laser is well stabilized, then the pulse train creates a spectrum consisting of narrow spikes at integer multiples of the repetition rate of the laser, or Æ’rep. This spectrum is called a frequency "comb" because it resembles a hair comb.
The pulses emitted by a frequency comb lasers are not identical. φCE is the small difference between the peak of the envelope and the peak of the light wave. φCE varies from pulse to pulse because the carrier wave and the envelope travel at different speeds inside the laser. This pulse-to-pulse change in φCE causes a small frequency shift in the entire comb by a specific amount ƒ0, also known as the comb offset frequency. Like the repetition rate (ƒrep), the comb offset frequency is determined by the specific characteristics of an individual mode-locked laser. The measurement of these two parameters makes it possible to completely characterize a frequency comb in terms of both time and frequency and determine the evolution of φCE, or ΔφCE.
NIST scientists also have demonstrated the most precise synthesis ever of optical frequencies, generating specific colors with a reproducibility of 19 digits. The experiments are a significant step toward next-generation "atomic clocks" based on optical rather than microwave frequencies.
NIST staff and collaborators also have extended the reach of frequency combs. One project extended the wavelength coverage 1,000 nanometers (a measure for wavelengths of light) farther into the infrared than ever before, while another effort at JILA created the world's first frequency comb in the extreme ultraviolet. In addition, NIST has shown that extremely stable microwave signals can be generated from optical frequency combs.
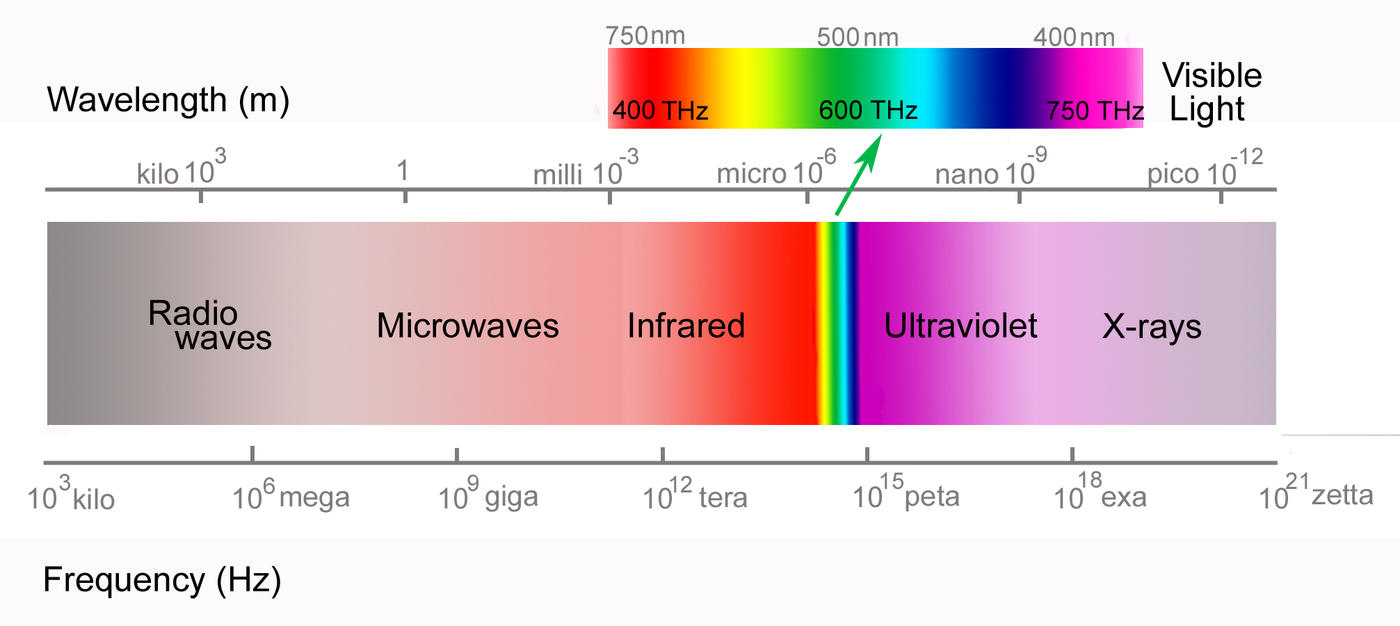
An optical "frequency comb" is a very precise tool for measuring different colors or frequencies of light.Now technology is able to measure the colors & frequency of optical fiber also. This technology also used in telecom sectors.The technology, made possible by recent advances in ultrafast lasers, can accurately measure much higher frequencies than any other tool. Frequency combs are already widely used in metrology laboratories and physics research, and they are starting to become commercially available.
Optical frequency combs rely on the relationship between time obviously a familiar concept and frequency, which is less familiar to most people, but is simply the number of oscillations per unit of time. NIST scientists start with lasers that emit a continuous train of very brief, closely spaced pulses of light containing a million different colors. The properties of the light over time are converted to frequency numbers to make what looks like a comb. Time and frequency are inversely related; that is, smaller units of time (or faster oscillations of light waves) result in larger frequency numbers.Optical frequency combs are central to the development of optical atomic clocks. Researchers are currently working on applications of combs in advanced laser radar, exquisitely sensitive chemical detectors, and secure optical communications systems. Laboratory scientists are using them to measure the energy levels of electrons and probe their dynamics inside atoms. And, thanks to frequency combs, revolutionary advances in our ability to exactly control chemical reactions with lasers are just around the corner.
The graphic below shows how a few different colors of light oscillate over time. This example is greatly simplified, and the specific units are unimportant (in reality the units would be tiny fractions of seconds). The essential point is that the blue waves oscillate much faster than the red waves, and the yellow and green waves are somewhere in between.
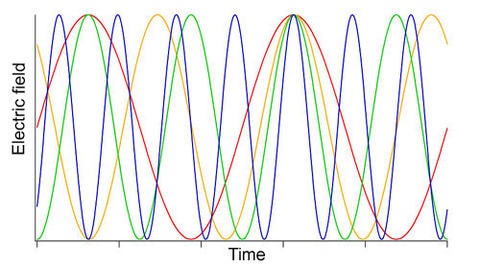
In the past few years, researchers have even used frequency combs to span the huge frequency (and wavelength) gap between radio and light waves, connecting them in ways that were impossible until just a few years ago. This connection goes to the heart of our ability to measure frequency and time, enabling the developing of a new generation of atomic clocks based on optical transitions in atoms. These new clocks use frequency combs to count the oscillations of light produced by optical atomic transitions and convert them to useful electronic signals. The comb technology used in optical atomic clocks is so precise that every one of the colors in a laser pulse train can be exactly linked with one another to produce (and measure) a symphony of hundreds of thousands of pure optical tones.
A simplified graphic of a corresponding frequency comb is shown below. Each "tooth" of the comb is a different color, arranged according to how fast the light wave oscillates in time. The waves that oscillate slowly (red) are on the left and the waves that oscillate faster (blue) are on the right. Frequency is measured in hertz, or cycles per second. An actual optical comb does not begin at zero on left, but at a very high number, 300 trillion hertz.
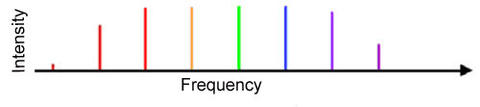
A real optical frequency comb spans the entire visible spectrum of light, and has very fine, evenly spaced teeth. The teeth can be used like a ruler to measure the light emitted by lasers, atoms, stars, or other objects with extraordinarily high precision.
Mode-locking refers to how the laser light is formed into pulses. In all lasers, light is repeatedly reflected within a mirrored cavity. In a mode-locked laser, the peaks of the different colors of light waves coincide at regular intervals, evenly spaced in time. The peaks build on each other to form very short, bright bursts of light, each containing many different frequencies
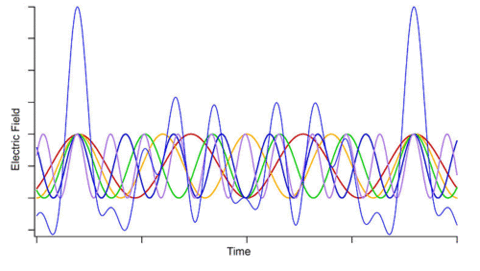
The timing between pulses determines the spacing between the teeth of the frequency comb. NIST scientists use lasers that emit about 1 billion pulses per second. The faster the pulse repetition rate, the wider the spacing between the teeth, making each individual tooth easier to identify.
Finally, the stability of the laser determines the width of the individual comb teeth. A highly stabilized laser produces very fine teeth, enabling highly precise measurements of specific frequencies or changes in frequency. Special crystals, mirrors, and other techniques also are used to make the light waves and comb teeth as perfectly spaced as possible.
How do Frequency Combs Work?
Optical frequency combs are particularly useful because the optical frequencies of comb lines can be determined by two radio frequencies, the repetition rate, and the offset frequency. High-speed photodiodes can readily measure the repetition rate. However, it wasn’t until 2000 that scientists at JILA figured out how to measure the offset frequency in a frequency comb that spanned an entire octave of frequencies. The octave-spanning spectrum allowed them to compare two comb lines at opposite ends of the spectrum with each other. If the offset frequency had been zero, then the two comb lines would have been found to have a frequency ratio of exactly 2. Instead, they found a small deviation, equal to the offset frequency, from this exact ideal ratio.
It turns out that the frequencies of two comb lines with a ratio of approximately 2 can be compared by doubling the frequency of the comb line on the low-frequency side of the spectrum. Frequency doubling is accomplished with second-harmonic generation crystals, which produce an output electric field that is the square of the input field. And, squaring a sine wave produces a sine wave at twice the frequency.
If the low frequency comb line has a frequency of νn = n•Æ’rep + Æ’0, then the comb line that is closest in frequency to twice νn has a frequency ν2n = 2n•Æ’rep + Æ’0. One can easily determine their frequency difference via a beat note that occurs when both illuminate the same photodetector (known as heterodyne detection, identical in concept to the "beating" sound used in tuning a musical instrument). This simple technique yields 2ν2n-νn = 2(n•Æ’rep + Æ’0) – (2n•Æ’rep + Æ’0) = Æ’0 and is known as "self-referencing." The output of heterodyne detection gives a signal that can be used to generate an error signal in a phase-locked feedback loop to stabilize the frequencies of all of the comb lines.
Frequency combs have dramatically simplified and improved the accuracy of frequency metrology. They also are making it possible to build optical atomic clocks, expected to be as much as 100 times more accurate than today's best time-keeping systems. Better clocks will lead to studies of, for example, the stability of the constants of nature over time, and enable improved technology for advanced communications and precision navigation systems, such as next-generation global positioning systems.
Highly accurate measurements of frequencies are also essential for many other advanced fields of science that require the identification or manipulation of atoms or molecules, such as detection of toxic biochemical agents, studies of ultrafast dynamics and quantum computing. As scientists continue to improve frequency comb technology and make it easier to use, it may be applied in many other research fields and technologies, from medical tests in doctor's offices, to synchronization of advanced telecommunications systems, to remote detection and range measurements for manufacturing or defense applications.
What Are Frequency Combs Made
Mode-locked lasers generate optical frequency combs by creating regular trains of incredibly short pulses of light that last anywhere from 20 to 100 quadrillionths of a second (10-15 s), depending on the individual laser. Today, well-stabilized mode-locked lasers, together with associated electronics and a specialized interferometer for comparing comb lines, are sometimes referred to as "Frequency Comb Lasers" or simply "Optical Frequency Combs." If such a laser is well stabilized, then the pulse train creates a spectrum consisting of narrow spikes at integer multiples of the repetition rate of the laser, or Æ’rep. This spectrum is called a frequency "comb" because it resembles a hair comb.
The pulses emitted by a frequency comb lasers are not identical. φCE is the small difference between the peak of the envelope and the peak of the light wave. φCE varies from pulse to pulse because the carrier wave and the envelope travel at different speeds inside the laser. This pulse-to-pulse change in φCE causes a small frequency shift in the entire comb by a specific amount ƒ0, also known as the comb offset frequency. Like the repetition rate (ƒrep), the comb offset frequency is determined by the specific characteristics of an individual mode-locked laser. The measurement of these two parameters makes it possible to completely characterize a frequency comb in terms of both time and frequency and determine the evolution of φCE, or ΔφCE.
NIST scientists also have demonstrated the most precise synthesis ever of optical frequencies, generating specific colors with a reproducibility of 19 digits. The experiments are a significant step toward next-generation "atomic clocks" based on optical rather than microwave frequencies.
NIST staff and collaborators also have extended the reach of frequency combs. One project extended the wavelength coverage 1,000 nanometers (a measure for wavelengths of light) farther into the infrared than ever before, while another effort at JILA created the world's first frequency comb in the extreme ultraviolet. In addition, NIST has shown that extremely stable microwave signals can be generated from optical frequency combs.
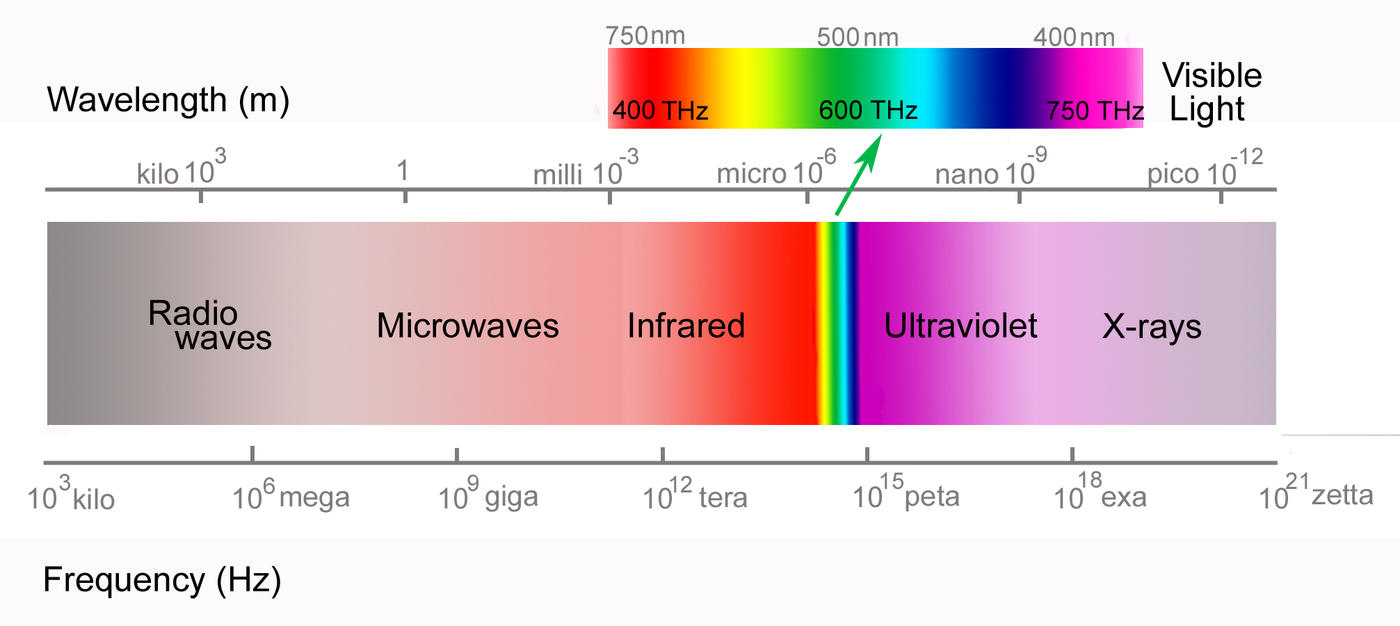
0 Comments